Blogue
Inventing 6G

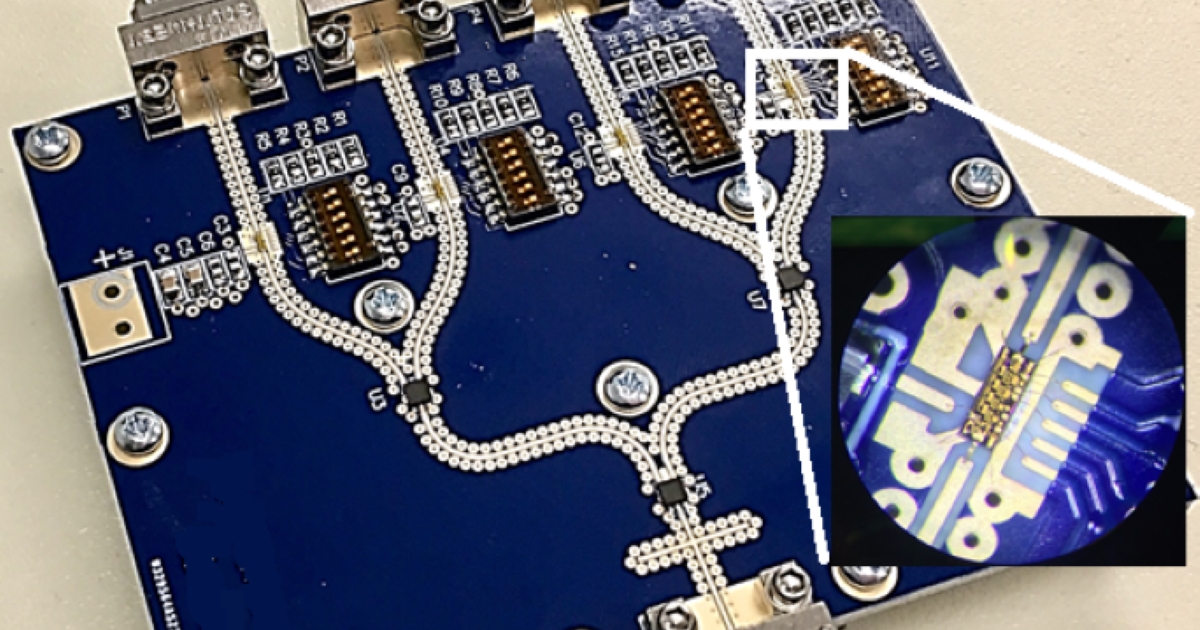
Mobile antennas in the 5G range are small, which makes them easy to integrate into electronic circuits; here they are on a millimeter-wave beamformer circuit. Newer 6G antennas will be even smaller. (Photo: Mohammad Sharawi)
While "5G" - aka the fifth generation - of standards for mobile telephony is currently being deployed in cities, engineers are working on the technology that will succeed it, “6G”. Professor Mohammad Sharawi is part of this group, and with his team, is developing and testing the next range of antennas. Antennas as tiny as the tip of a few strands of your hair!
Over the past 40 years, cellular device antennas have gradually become smaller and smaller. The first antennas that could host a long antenna of 30 centimeters, gave way to devices with shorter antennas. Today, these important elements are even more imperceptible, hidden behind the screen of smart phones.
You'd perhaps think there's an aesthetic reason behind this shrinkage, but in fact, no. If antennas are smaller today, it's above all so they're able to capture and emit waves at higher frequencies. So, the reason is physical, and we'll return to this later.
Microscopic antennas
![]() |
Each new generation of cell phones comes with a new frequency range, always higher in the band than previous ones. In the 1990s, antennas had to pick up waves around 900 megahertz (MHz). Today with 5G, they target frequencies ranging from 10 to 50 gigahertz (GHz), or 10,000 to 50,000 MHz, in the shortwave region of the electromagnetic spectrum.
And we haven't stopped climbing yet.
In his Polytechnique Montréal laboratory, Associate Professor Mohammad Sharawi from the Department of Electrical Engineering is developing the next generation of antennas. So where do these antennas transmit? In frequencies from 0.1 to 0.3 Terahertz (THz), or 100 000 to 300 000 MHz.
Why go after frequencies of another order of magnitude? “Simply because they make it possible to push a lot more data, more quickly,” explains Professor Sharawi.
![]() |
Until now, all cellular technologies have exploited frequencies located in a thin band of the electromagnetic spectrum, located between that of radio waves and that of microwaves. By targeting frequencies of the order of Terahertz, in a region of the spectrum corresponding to the far infrared, we get a little closer to light waves, and the transmission capacities of fiber optics.
"At these frequencies, we should reach transmission speeds of the order of one terabyte per second. “We'll open the door to new applications, in addition to improving those that rely on 5G, and that require instantaneous data exchange."
Among these applications and uses, the engineer mentions autonomous vehicle technology which is largely based on the exchange of data. “Higher transmission speeds will also make it possible to perform remote surgeries, or even fly an airplane remotely. The important thing is to reduce latency as much as possible."
Professor Sharawi notes that this next generation's antennas will be so small that they can be incorporated into integrated circuits or electronic chips. New ways of using antennas will also be considered, he adds. “For example, they'll be so small that we could integrate them into windowpane glass in order to facilitate the exchange of data entering and leaving buildings."
The basics... of antenna design |
Cell phone antennas play the role of both transmitter and receiver. When transmitting data, an antenna converts an electrical signal from the phone into electromagnetic waves. When receiving data, the reverse occurs. Before 4G and 5G technology, antennas were one of the biggest components in mobile devices - now they fit on the tip of your finger. Why? Because to work optimally, an antenna must be inversely proportional to the frequency it's targeting: the higher the frequency targeted, the shorter the antenna needs to be to pick up and transmit waves at that frequency. In reality, the major factor considered is wavelength, i.e., the distance between each wave's peaks, a variable inversely proportional to frequency. For an antenna to work optimally, it must be half the size of the wavelength it targets. For example, 900 MHz waves used by 2G (second generation) devices have a wavelength of 33.3 centimeters (cm). These devices' antennas had to be a little over 15 cm. The wavelength associated with a 30 GHz frequency is 1 cm. So, 5G antennas must therefore be about ... 0.5 cm long. |
additive manufacturing and optics
![]() |
Question: for 6G antennas that target around 0,3 THz, what size antenna will be required? Answer: well under a millimeter... about 0,5 millimeters, or 450 micrometers. That's the width of six hairs!
Since everyone eventually wants to reach 1 THz frequencies, some antennas could even be smaller in size...
"It's definately really tiny," Sharawi admits, without any discouragement. His team is relying on the same approaches used in microelectronics to make components of this size - layer by layer. Professor Sharawi collaborates with the Thin Film Physics and Technology Research Group (GCM) and is able to use their “clean room” in the J-Armand Bombardier Building. He’s also busy developing new ways to assemble multi-functional and encapsulated integrated antennas through additive manufacturing with the Multiscale Mechanics Laboratory (LM2).
“The challenge is to balance the functions of transmission and reception. At this size, anything can disrupt their operation," he adds.
The challenge is all the more important because these waves belong to the far-infrared group, waves that behave like light particles. "This forces us to work with a multidisciplinary approach," says Professor Sharawi. "We have to combine our knowledge of radio frequency technology with that of photonics."
Since 2019, his group has filed seven patent applications related to the development of these future antennas, or which propose original ways to test them. Still, a lot of work will still be needed, Professor Sharawi points out, “This technology is still far from being ready."
Learn more
Professor Mohammad Sharawi's expertise
Departement of electrical engineering website
Poly-Grames website
Groupe de recherche en physique et technologie des couches minces (GCM) website
Laboratoire de mécanique multi-échelles (LM2) website
Comments
Commenter
* champs obligatoire